수직점프 수행에 있어서 근육과 건의 상호작용의 역할
The Role of Muscle-Tendon Interaction in Vertical Jumping
Article information
Abstract
본 연구에서는 많은 스포츠 활동에서 기초적으로 갖추어야하는 수직 점프(vertical jump) 동작의 생체역학에 대하여 최신 연구 동행을 바탕으로 고찰해 보았다. 수직 점프 능력의 극대화를 위해서는 기본적으로 짧은 시간에 큰 힘을 발현할 수 있는 능력을 필요로 한다. 이를 위하여, 다분절 인체 구조의 효율적인 협응과 더불어, 바이오 엔진이라고 할 수 있는 근-건 복합체(muscle-tendon complx, MTC)내 근육과 건의 상호작용의 역할은 핵심적이라고 할 수 있다. 특히 수축 속도로 인한 제약으로 근육의 힘 발현이 제한되어 있는 점을 고려 할 때 근육과 물리적으로 직렬 연결된 탄성요소의 신장 및 탄성복원을 통한 파워 증폭 메커니즘(Astley & Roberts, 2014)에 특히 초점을 두고 고찰하였다. 본 연구를 통하여 기초적인 스포츠 동작중 하나인 점프 동작 메커니즘에 대한 깊이 있는 이해와 다분야 융합연구 촉진을 도모하고자 하였다.
Trans Abstract
Vertical jumping is one of basic skills in many sports activities. Maximizing vertical jumping performance requires large “power”, which implies that one should generate force against the ground in a short period of time. In order to gain better understandings of how human musculo-skeletal system mechanically functions to achieve maximal power in vertical jumping, the proposed “dynamic catch” mechanism, one of “power amplification” mechanisms through the role of muscle-tendon interaction, was specifically reviewed base on the morphological and mechanical characteristics of lower limb muscle-tendon complex. By understanding basic structural and functional features of human muscle-tendon interaction, this review aims to provide basic scientific information for training and rehabilitation and promote convergence researches in related areas, such as sports biomechanics, mechanical engineering, and sports medicine.
서 론
인체의 움직임은 기본적으로 골격근(muscle)이 발현하는 기계학적인 힘이 건(tendon)을 통해서 뼈에 전달되고, 연결된 뼈들로 구성된 관절(joint)의 움직임을 유발하여 이루어진다고 볼 수 있다. 물론 근육이 발현하는 능동적인 힘(active force)과 외부에서 인체에 작용하는 힘(external forces)도 중요한 요소이기도 하다. 특히 힘 생성과 전달에 관한 여러 가지 요인 가운데 근육과 건으로 구성된 기능적 단위인 근-건 복합체(MTC)내에서 근육과 건의 유기적인 상호 작용을 통한 에너지 보존(energy conservation), 파워 증폭(power amplification), 파워 상쇄(power attenuation)와 같은 다양한 기능(Roberts & Azizi, 2011)을 보면 우리 인체의 스마트한 디자인에 놀라지 않을 수 없다.
특히 작은 부하의 일상적인 움직임을 수행할 때와 달리 많은 스포츠 활동에서 요구되는 기초 기능이라고 할 수 있는 점프(jump) 동작에서는 순간적으로 폭발적인 파워를 요구하기 때문에 기계학적인 힘을 발현하는 골격근의 역할과 더불어 탄성을 가지고 직렬로 연결된 건의 역할이 중요하다고 알려져 있어서(Alexander, 2002) 근-건 복합체의 파워 증폭 메커니즘에 연구자들의 많은 관심이 주목되고 있다.
근-건 복합체 내에서 근육과 건의 상호작용을 통한 파워 증폭 메커니즘에 대한 대표적 연구로는, 곤충과 동물을 대상으로 한 연구(Bennet-Clark, 1975; Alexander & Bennet-Clark, 1977; Roberts & Marsh, 2003; Astley & Roberts, 2012) 뿐만 아니라 인체를 대상으로 한 시뮬레이션 연구(Bobbert, 2001), 그리고 생체(in vivo) 실험 연구(Kurokawa et al., 2001; Farris et al., 2016)등과 같이 다양하게 수행되고 있다.
근건 복합체의 다양한 상호작용에 대한 연구는 많은 연구자들의 관심이자 전공자들에게는 필수적인 지식요소이므로 이 시점에서 지금까지 발전되어 온 관련한 이론, 연구분야, 최신 경향, 앞으로의 연구 방향 등 다양한 관점에서 정리해 보는 것은 의미가 있다. 따라서 본 연구에서는 수직점프 수행 시, 근육과 건의 상호작용에 의한 파워 증폭 메커니즘에 대하여 근-건 복합체의 구조적, 기계학적 특성 및 인체 분절의 협응동작을 바탕으로 고찰하고자 한다. 이를 통하여 궁극적으로 기초적인 인체 동작인 수직점프에서 근-건 복합체의 구조-기능에 대한 최신 연구 정보를 공유하고, 더 나아가 운동역학, 재활의학, 기계공학 등 유관 연구 분야 간의 융합연구 활성화를 위한 기반을 마련하고자 한다.
수직점프
점프는 “몸을 날리어 높은 곳으로 오름” 이라고 정의된다(표준국어대사전, 국립국어원). 점프는 다양한 스포츠 종목에서 기초가 되며, 수직 혹은 수평 방향으로 최대한 거리에 도달하고자 수행하는 동작이라고 할 수 있다. 다양한 형태의 점프 중에 가장 단순하다고 할 수 있는 수직점프(vertical jump)는 “도움닫기를 하지 아니하고 그 자리에서 수직으로 점프하는 일”로 정의되며(표준국어대사전, 국립국어원), 전신 체력을 측정하는데 사용되기도 한다.
기본적으로 수직점프 수행을 위해서는 순간적으로 큰 힘을 발현할 수 있는 근신경계의 기능과 더불어 인체 분절을 효율적으로 움직일 수 있는 협응 능력을 필요로 한다. 이러한 수직점프 동작을 분석하고자 할 때 대항운동(countermovement) 구간, 추진(propulsion) 구간, 비행(flight) 구간, 착지(landing) 구간<Table 1>으로 나누어 분석하는 것이 일반적이며, 최대 수직점프 수행 결과는 대항운동 구간과 추진 구간에서 인체 분절의 협응, 근-건 복합체 내에서 근육의 근력 발현 특성과 직렬 탄성요소(SEE)의 특성 및 상호작용이 수행 결과에 중요한 영향을 미친다고 할 수 있다.
대항운동 구간은 점프하는 반대 방향으로의 움직임이 일어나는 구간으로, 엉덩관절, 무릎관절, 발목관절이 굴전(flexion)되어 추진 구간에서 보다 큰 운동 범위를 확보하게 되고 이를 통해 보다 효율적으로 추진력을 얻을 수 있는 자세를 확보하게 된다. 더불어 이 구간에서 엉덩 관절, 무릎 관절, 발목 관절의 굴전은 추진 구간에서 동원되어 단축성 수축(shortening 혹은 concentric contraction)을 수행하게 되는 신전근들을 자연스럽게 사전 신전(pre-stretch) 시키게 되어 단축성 수축 직전에 신장성 수축이 선행되는 신전-단축 주기(SSC) 효과를 유도하게 되고 추진 구간의 효율성을 향상 시키게 된다고 알려져 있다(Komi & Bosco, 1978; Bosco et al., 1982; Svantesson et al., 1994).
수직점프의 유형과 관련하여, 대항운동의 유무(즉, countermovement jump vs. squat jump)가 수직점프 수행 결과에 미치는 영향에 관해서는 대항운동이 포함된 수직점프가 약 10% 정도 우수한 것으로 알려져 있다(Bobbert et al., 1996; Earp et al., 2010; Earp et al., 2011). 그 이유로는 대항운동이 없는 경우 추진 구간에서 근육의 활성화와 발현되는 근력이 최대값에 미치지 못하거나(Bobbert et al.,1996; Zajac 1993), 탄성 에너지 이용이 상대적으로 낮기 때문(Komi & Bosco, 1978)이라고 설명되고 있다.
수직점프 수행 시 근-건 복합체 내에서 탄성 에너지 활용과 관련하여, Alexander(1995)의 연구에서는 대항운동이 있는 경우 탄성 에너지의 우세한 활용으로 인해 수직점프 수행 결과를 향상 시킨다고 보고한 반면, Bobbert et al.(1996)와 Anderson & Pandy(1993), 그리고 Farris et al.(2016)의 연구에서는 두 유형의 수직점프에서 직렬 탄성요소의 동원 방식에 차이가 있을 뿐 수직점프 수행 결과에 미치는 영향은 미미하다는 견해를 보이고 있다. 하지만 대항운동의 유무와 별개로 모든 유형의 점프 동작에서 근육자체가 발현할 수 있는 파워보다 더 큰 파워가 발현된다는 점(Roberts & Azizi, 2011; Roberts & Marsh, 2003)을 고려할 때 근-건 복합체 내의 근육과 건의 상호 작용 및 탄성 에너지를 활용한 메커니즘은 많은 연구자들의 지속적인 관심사로 남아 있다.
근-건 복합체
거시 해부학
수직점프 동작을 수행하는데 있어서 주된 역할을 하는 인체의 하지 근육들은 대부분 탄성 특성을 갖는 건조직을 통해 뼈에 연결되어 있다. 상대적으로 큰 근량으로 파워 발현에 큰 역할을 하는 엉덩관절의 신전근들도 있지만(Ward et al., 2002), 먼쪽(distal)으로 갈수록 근육의 크기는 작아지고 보다 긴 건조직과 연결되어 탄성 에너지 활용에 유리한 특성을 보이고 있다. 무릎신전근은 무릎인대(patellar tendon)와 발목 발바닥쪽굽힘근의 경우는 발꿈치힘줄(achilles tendon)과 같은 상당히 긴 건 조직을 통해 연결되어 있다<Fig. 1>.

Knee extensor (top) and ankle plantarflexor(bottom)
(Muscle Premium, Version 3.1.3, VisibleBody, Argosy Publishing Inc.)
특히 수직점프 수행 시 추진구간의 마지막에 파워 증폭에 큰 기여를 하는 것으로 알려진 발목관절의 발바닥쪽굽힘근의 경우, 원형 코드 형태의 발꿈치힘줄 뿐만 아니라 가자미근(soleus)과 장딴지근(gastrocnemius)의 근다발(fascicle)이 연결되는 판 형태의 힘줄(aponeurosis)이 복잡한 형태로 근육 내에 자리 잡고 있다(Hodgson et al., 2006; Lee, et al., 2006)<Fig. 2>.
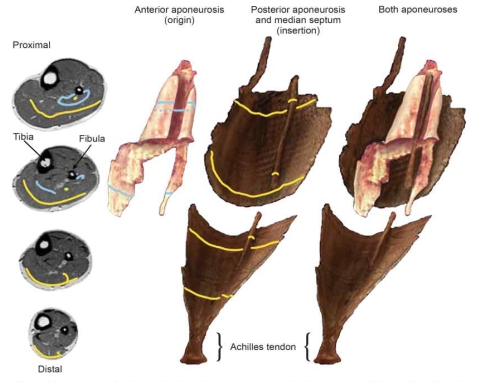
Tendinous structure in the ankle plantar flexor muscle-tendon complex in human (Hodgson, et al., 2006)
탄성요소
생체역학적 측면에서 근육의 거시적 구조를 보면, 탄성을 갖는 많은 구조를 찾아 볼 수 있다. 앞서 언급한 거시적인 측면에서 보면 대표적으로 건 조직과 근육을 에워싸고 있는 근막(fascia)과 근육바깥막(epimysium), 근섬유다발을 싸고 있는 근다발막(perimysium), 근섬유를 싸고 있는 근섬유막(endomysium)이 있다. 미시적 측면에서 보면 교차결합(cross-bridge) 내의 탄성요소(Huxley & Simmons, 1971), 최근 주목을 받고 있는 티틴(titin)과 세포외 기질(extracellular matrix, ECM)에 포함된 단백질 구조(Roberts, 2016)를 포함시킬 수 있다.
생체역학에서 사용되는 대표적인 근육 모델은 근육의 힘 발현 예측을 목적으로 하는 현상학적 Hill-type 모델(Hill, 1938)과 근육의 미시적 구조 수준에서 힘 발현 메커니즘 규명에 초점을 맞춘 구조 기반 Cross-bridge 모델(Huxly, 1957; Huxley & Simmons, 1971)을 들 수 있는데, 이 모델들에는 능동적인 힘(active force)을 발현하는 수축요소(CE)와 단순한 스프링 형태로 수동적인 힘(passive force)을 발현할 수 있는 직렬/병렬 탄성요소(SEE와 PEE)가 포함되어 있다. Hill-type 모델의 경우 일반적으로 근육에 연결된 무릎인대와 발꿈치힘줄과 같은 건 조직을 직렬 탄성요소로 고려하고 있으며, Cross-bridge 모델의 경우 교차결합을 구성하는 마이오신 필라멘트(myosin filament) 내에(Huxley & Simmons, 1971) 존재한다고 보고 있다. 더불어 근 수축 단백질 구조인 액틴 필라멘트(actin filament)와 마이오신 필라멘트(Wakayabashi, 1994), 그리고 최근 주목 받고 있는 제 3의 근수축 관련 단백질 구조인 티틴(titin)(Linke, 2000) 자체에도 탄성 특성이 존재한다고 보고되고 있다.
건 조직
본 연구의 초점인 건은 근육과 직렬로 연결되어 근-건 복합체 내에서 근육과의 상호작용을 통해 기능적으로 중요한 역할을 수행하고 있다는 점에서 주목을 받고 있다. 건은 외력이 가해지면 그 길이가 늘어나고 그 힘이 사라지면 원래의 길이로 복원하는 전형적인 탄성 특성을 가지고 있다<Fig. 3>. 최근 초음파영상기법을 사용해 생체조건 하에서 인체의 건 특성을 최대 등척성 수축 시 살펴 본 대표적인 연구의 결과는 아래와 같다.

Tendon stress-strain curve(Wang, 2006)
Maganaris & Paul(2002)의 연구에서 측정한 인체 장딴지근에 연결된 건의 탄성 경우, 건은 87.5 ± 8.5 N의 힘에 1.7 ± 1 mm(0.8 ± 0.3%), 875 ± 85 N의 힘에 11.1 ± 3.1 mm(4.9 ± 1%)의 변형률(strain)을 보였다. Young의 계수(Young’s modulus)와 이력현상(hysteresis)은 1.16 ± 0.15 GPa과 18 ± 3%를 보인다고 보고되고 있다.
Hansen et al.(2007)의 연구에서 측정한 무릎인대(patella tendon)의 경우, 6.9 ± 0.6%의 변형률을 보였으며, 4334 ± 562 N/mm의 강성(stiffness)과 1.09 ± 0.12 GPa의 Young의 계수를 관찰하였다.
Magnusson et al.(2001)의 연구에서 살펴 본 발꿈치힘줄(Achilles tendon)의 경우, 4.4~5.6%의 변형률과 1.048~1.474 GPa의 Young의 계수를 관찰하였다.
근-건 상호작용의 역할
탄성 특성을 가지고 근육과 직렬로 연결된 건 조직은 근육이 발현하는 힘과 외력에 의해 신장되며, 이 힘이 사라지면 신장 시에 건 조직에 저장된 탄성 에너지를 방출하여 동작의 효율성에 활용하는 것으로 알려져 있다(Alxander, 2002). 그러나 이와 같은 현상을 수직점프 수행과 연관시켜 어떤 메커니즘에 의해서 건의 탄성 특성이 동작의 효율성을 향상 시키는지에 대해 다양한 의견이 제시되고 있다.
놀라운 점프 능력을 보이는 곤충과 개구리목 동물에 대한 연구(Bennet-Clark, 1975; Alexander & Bennet-Clark, 1977; Roberts & Marsh, 2003; Astley & Roberts, 2012)와 더불어 인간의 점프 수행 능력에 대한 실험연구(Bohm et al., 2006; Farris et al., 2016; Finni et al., 2000; Fukashiro et al., 1993; Gollhofer et al., 1992; Hof et al., 2002; Kiehn & Eken, 1997; Kurokawa et al., 2001; Spagele et al., 1999)와 시뮬레이션 연구(Bohm et al., 2006; Finni et al., 2000; Kurokawa et al., 2001; Spagele et al., 1999)가 수행되어 왔다.
특히 직렬 탄성요소의 활용에 의한 파워 증폭을 설명하기 위해서(1) 중력에 의한 부하(gravitational loading) (Farris et al., 2016; Bobbert et al., 1996; Anderson & Pandy, 1993),(2) 관절역학(joint kinetics) (Bobbert & van Ingen Schenau, 1988; Astley & Roberts, 2014),(3) 유효 기계적 증폭률(effective mechanical advantage, EMA)(Roberts & Marsh, 2003; Astley & Roberts, 2014), 그리고(4) 다관절 근육의 기여(biarticular muscle contribution)(Farris et al., 2016)와 같은 메커니즘들이 제시되어 있다.
현재 제안된 메커니즘들의 중심에는 “투석기 메커니즘(catapult mechanism)”(Roberts & Azizi, 2011)이 핵심적이라고 할 수 있다. 즉 인간을 포함한 척추동물에서는 점프 동작 직전, 즉 대항운동 구간에서 수축 요소가 발현하는 힘에 의해서 서서히 직렬 탄성요소가 신장되고, 점프 동작의 추진 구간에서 신장된 직렬 탄성요소가 늘어난 고무줄이 원래의 길이로 복원되는 현상(탄성복원, recoil)과 같이 빠른 속도로 짧아지면서 근육 자체만이 작동했을 때와 비교하여 보다 큰 파워로 점프를 수행할 수 있다고 그 원리를 설명하고 있다. 이와 같은 원리에 의해서 근육은 좀 더 느린 속도로 수축이 가능하여, 힘-속도 관계에 비추어 더 큰 힘을 생산하고, 따라서 더 많은 기계학적 일을 수행할 수 있다(Bobbert, 2001; Roberts & Azizi, 2011).
중력 부하와 SEE 신장
대항운동 구간의 유무에 상관없이 수직점프를 수행 할 때, 추진 구간의 시작 순간 엉덩관절, 무릎관절, 발목관절은 추진력을 발현하기 위한 스쿼트 자세를 취하게 된다. 대항운동 구간이 없는 스쿼트 점프를 관찰한 Farris et al.(2016)의 연구 결과에 따르면, 추진 구간 초기 스쿼트 자세에서 상향 움직임이 시작 되어 SEE의 탄성복원이 나타나는 시점까지의 구간에서 가자미근 SEE는 1.0 mm, 장딴지근 SEE는 약 5.8 mm의 신장이 관찰되었으며, 이는 전체 탄성복원이 가자미근 SEE는 39.3 mm, 장딴지근 SEE는 12.3 mm로 관찰된 점에 비추어, 추진 구간 시작 시점에서 가자미근 SEE는 97%, 장딴지근 SEE는 53% 사전 신장 되어 있었음을 알수 있다고 보고, 이는 중력(체중)이 SEE의 신장에 기여하는 중요한 요인이라는 주장을 뒷받침 하였다. 더불어 장딴지세갈래근(triceps surae)의 근활성도와 발목 관절 모멘트는 점프구간에서 도달한 최대치에 50% 이상 도달했다는 점은 중력에 의한 장딴지세갈래근 SEE의 신장 및 탄성복원 기여 메커니즘을 뒷받침 한다고 보고 하였다.
부가적으로 대항운동 구간의 유무(즉, countermovement jump vs. squat jump)에 따른 수직 점프의 수행 결과에서 대항운동 구간이 있는 점프가 수행 결과가 우수하지만(Anderson & Pandy, 1993; Bobbert et al., 1996), 시뮬레이션 연구 결과, SEE의 신장 및 탄성복원의 기여도는 결론적으로 수행 결과 차이에 큰 기여를 하지 못하며, 수행 결과의 차이는 증가된 근활성화 및 근력 발현 역학에 기인한다고 볼 수 있다(Bobbert et al., 1996). 하지만 중력에 의한 SEE의 신장 및 탄성 복원의 기여는 두 점프 형태에서 모두 긍정적이며, 다만 대항운동 구간이 있는 점프에서는 대항운동 구간에서 위치에너지의 감소(체중에 의한 지면 반력보다 수직 지면반력 값이 낮아지는 negative work 구간)가, 대항운동 구간이 없는 점프에서는 근력 에 의한 SEE의 신장 및 탄성복원의 원천이라고 할 수 있다(Anderson & Pandy, 1993).
관절역학과 SEE 신장
수직 점프를 분석하는데 있어서 다양한 관절과 분절의 움직임을 관찰할 수 있다. 팔의 움직임을 포함시켜 관찰하는 경우도 있지만, 일반적으로 머리, 팔, 몸통을 하나의 분절로 보고, 엉덩관절, 무릎관절, 발목관절로 단순화하여 분석하는 것이 일반적이다. 이 분절과 관절이 어떤 패턴으로 동작을 수행하는가, 즉 협응(coordination)도 수직 점프 수행의 결과에 영향을 미치는 핵심 요인으로 분석되고 있다. 인체(Bobbert & van Ingen Schenau, 1988; Farris et al, 2016)와 동물(Astley & Roberts, 2014) 연구를 통해서 수직 점프 수행에 있어서 먼쪽(distal) 관절의 움직임이 추진 구간 후반부로 지연될수록 수행 결과가 향상됨을 확인할 수 있다. 이 메커니즘에 의한 SEE의 신장 및 탄성복원 기여는, 몸쪽(proximal) 관절(엉덩관절과 무릎관절)이 먼쪽 관절의 움직임에 선행하여 순차적으로 작동할 경우, 몸통 분절을 우선적으로 가속하고, 수직 지면반력을 증가시키는 결과를 가져온다. 추진 구간에서 이렇게 발현되는 체중을 초과하는 증가된 지면 반력 때문에 먼쪽 관절(발목관절)은 저항하게 되고(동작 지연), 결과적으로 이 과정에서 근육의 활성도 증가와 함께 상응하는 근력 발현 증가가 나타나게 되는데, 이로 인해 SEE의 신장을 유발하여 탄성 에너지를 저장할 수 있게 되고, 추진 구간 말미 탄성복원에 사용할 수 있도록 하는 결과를 가져오게 된다는 것이 관절역학에 의한 SEE 신장-탄성복원 메커니즘이다.
유효 기계적 증폭률(EMA)과 SEE 신장
유효 기계적 증폭률(effective mechanical advantage, EMA)은 기계적 장치 혹은 도구를 사용하여 얻을 수 있는 입력 힘 대비 출력 힘의 증폭 비율로 정의 할 수 있다<Eq. 1>. 쉬운 예로는 지렛대의 원리로, 적은 힘과 긴 모멘트 암 길이로 큰 힘을 얻을 수 있는 시스템에서 입력 힘 대비 출력 힘의 비율을 말한다.
수직 점프 수행 시 측정된 파워가 근육만이 작동했을 때 발현 할 수 있는 파워 보다 초과되는 현상이 동물 연구(Roberts & Marsh, 2003; Pelplowski & Marsh, 1997)와 인체 연구(Farris et al., 2016)에서 지속적으로 관찰되어 왔다. 초과한 점프 파워의 원천으로는 동작 수행 중 근-건 복합체 내의 SEE가 탄성 에너지를 저장하여, 근육과 달리 속도에 영향을 받지 않고, 빠르게 방출할 수 있기 때문이라고 설명되고 있다(Marsh, 1994, 1999). 이와 같은 SEE의 신장과 탄성복원 역할을 설명하는 또 다른 하나의 메커니즘으로 Roberts & Marsh(2003)이 제안한 가변 EMS 메커니즘이 있다. 경이로운 점프 능력을 보이는 곤충의 경우 투석기 메커니즘(catapult mechanism)을 이용하기 위해 미세한 에너지로 지속적 근수축 상태를 유지하는 제동메커니즘(catch mechanism, 생명과학대사전, 2014)을 사용하여 SEE를 신장시켜 근파워를 증폭시키는 것으로 알려져 있다(Bennet-Clark & Lucey, 1967; Bennet-Clark, 1975). 하지만 동물(개구리)의 경우 제동 메커니즘이 없기 때문에 유효 기계학적 증폭률의 조절을 통해서 유사한 효과를 얻을 수 있다(동적 제동 메커니즘(dynamic catch mechanism); Roberts & Marsh, 2003; Astley & Roberts, 2014). 즉, 추진 구간 초기 EMA의 증가 후 감소가 마치 곤충에서 관찰된 제동 메커니즘의 형태로 작동하였을 것이라 추정되었지만 이 메커니즘은 인체에서는 작동하지 않는 것으로 관찰되었다(Farris et al., 2016). Farris et al.(2016)의 인간의 스쿼트 점프를 대상으로 한 연구에서 추진 구간 초기 EMA가 최대값을 보였고, 상향 동작이 진행될수록 EMA의 선형 감소를 보여 인체에서는 개구리와 같은 동적 제동 메커니즘은 유효하지 않다는 점을 제시하였다.
다관절 근육의 기여와 SEE 신장
최근 Farris et al.(2016)는 인체 스쿼트 점프를 대상으로 발목관절 발바닥쪽굽힘의 주동근인 장딴지세갈래근 MTC의 길이 변화 패턴을 세밀하게 분석하였다. 특히 다관절 근육인 장딴지근과 단관절 근육인 가자미근의 독립적인 움직임, 즉 장딴지근과 가자미근의 근건 복함체 상호작용이 상이함을 관찰하였다. 가자미근 MTC의 경우, 발목 관절의 회전이 시작되는 시점부터 take-off 시점까지 점진적으로 그 길이가 짧아진 반면, 장딴지근 MTC의 경우 추진 구간의 약 60%까지 약간의 신장이 나타난 것을 관찰 하였다. 장딴지근 MTC의 길이 변화 패턴은 Bobbert et al.(1986)의 연구 결과와 유사하며, 이 관찰을 통해서 다관절 근육인 장딴지근 MTC가 무릎관절의 신전에 의해 발현되는 파워를 먼쪽 관절인 발목관절에 전달하는 역할뿐만 아니라 추진구간 전반에서 근섬유의 단축에 다른 추가적인 SEE의 신장(5.8 mm)을 유도하여 추가적인 파워 증폭에 기여하는 메커니즘으로 작용하지 않을까 제안하였다.
결론 및 제언
본 연구에서는 많은 스포츠 동작에서 기초가 되는 기본적인 능력인 점프 동작의 생체역학적 메커니즘에 대하여 최신 연구 동향을 바탕으로 알아보고자 하였다.
점프 동작 수행을 위하여 순간적으로 큰 파워를 발현하기 위해 다분절로 이루어진 인체의 조화로운 협응과 더불어 인체의 바이오 엔진이라고 할 수 있는 근-건 복합체의 상호작용, 특히 근육과 물리적으로 직렬 연결된 탄성요소의 신전 및 탄성복원을 통한 파워 증폭 메커니즘은 근육의 수축 속도로 인한 제한적인 힘 발현 능력을 증폭시키는 중요한 역할을 수행한다는 점을 명확히 알 수 있었다.
본 연구에서 고찰한 점프 수행의 기초적인 메커니즘에 대한 이해는 동작 자체에 대해 깊이 있는 이해를 도모할 수 있다고 판단되며, 이를 바탕으로 운동 수행 극대화를 위한 전문적인 트레이닝의 효과 분석, 운동 기능 복원을 위한 재활의 효과 검증, 다양한 스포츠 종목에서 요구되는 특성화 점프 요인 분석 등에 활용 될 수 있을 것으로 판단된다.
더불어 동물 및 인체를 대상으로 한 점프 수행 동작의 보다 깊이 있는 측정 및 분석을 위해서는 영상분석 장비, 근전도 측정 장비, 지면반력 측정 장비, 의료영상 장비 등 시스템의 첨단화가 필요하며, 더불어 모델링/시뮬레이션 연구를 위한 보다 정밀한 모델 개발 및 검증이 필요할 것으로 판단된다. 이를 위해서는 학문의 영역을 넘어 다각적이고 융합적인 접근 노력이 이 연구 분야의 발전에 핵심적인 역할을 할 것으로 믿어 의심치 않는다.
약어(Abbreviation)
MTC
muscle-tendon complex, 근-건 복합체
CE
contractile element, 수축요소
SEE
series elastic element, 직렬 탄성요소
PEE
parallel elastic element, 병렬 탄성요소
SSC
stretch-shortening cycle, 신전-단축 주기
EMA
effective mechanical advantage, 유효 기계적 증폭률
References
표준국어대사전, http://stdweb2.korean.go.kr/main.jsp, 국립국어원
. 표준국어대사전 http://stdweb2.korean.go.kr/main.jsp. 국립국어원.Alexander, R. M.(1995). Leg design and jumping technique for humans, other vertebrates and insects. Philosophical Transaction of the Royal Society London B Biological Sciences, 347(1321), 235-248.
Alexander R. M.. 1995;Leg design and jumping technique for humans, other vertebrates and insects. Philosophical Transaction of the Royal Society London B Biological Sciences 347(1321):235–248. 10.1098/rstb.1995.0024.Alexander, R. M.(2002). Tendon elasticity and muscle function. Comparative Biochemistry and Physiolology Part A: Molecular & Integrative Physiology, 133(4), 1001-1011.
Alexander R. M.. 2002;Tendon elasticity and muscle function. Comparative Biochemistry and Physiolology Part A: Molecular & Integrative Physiology 133(4):1001–1011.Alexander, R. M. & Bennet-Clark, H. C.(1977). Storage of elastic strain energy in muscle and other tissues. Nature, 265(5590), 114-117.
Alexander R. M., et al, Bennet-Clark H. C.. 1977;Storage of elastic strain energy in muscle and other tissues. Nature 265(5590):114–117. 10.1038/265114a0.Anderson, F. C. & Pandy, M. G.(1993). Storage and utilization of elastic strain energy during jumping. Journal of Biomechanics, 26(12), 1413-1427.
Anderson F. C., et al, Pandy M. G.. 1993;Storage and utilization of elastic strain energy during jumping. Journal of Biomechanics 26(12):1413–1427. 10.1016/0021-9290(93)90092-s.Astley, H. C. & Roberts, T. J.(2012). Evidence for a vertebrate catapult: elastic energy storage in the plantaris tendon during frog jumping. Biology Letter, 8(3), 386-389.
Astley H. C., et al, Roberts T. J.. 2012;Evidence for a vertebrate catapult: elastic energy storage in the plantaris tendon during frog jumping. Biology Letter 8(3):386–389. 10.1098/rsbl.2011.0982.Astley, H. C. & Roberts, T. J.(2014). The mechanics of elastic loading and recoil in anuran jumping. Journal of Experimental Biology, 217(24), 4372-4378.
Astley H. C., et al, Roberts T. J.. 2014;The mechanics of elastic loading and recoil in anuran jumping. Journal of Experimental Biology 217(24):4372–4378. 10.1242/jeb.110296.Bennet-Clark, H. C.(1975). The energetics of the jump of the locust Schistocerca gregaria. Journal of Experimental Biology, 63(1), 53-83.
Bennet-Clark H. C.. 1975;The energetics of the jump of the locust Schistocerca gregaria. Journal of Experimental Biology 63(1):53–83.Bennet-Clark, H. C. & Lucey, E. C.(1967). The jump of the flea: a study of the energetics and a model of the mechanism. Journal of Experimental Biology, 47(1), 59-67.
Bennet-Clark H. C., et al, Lucey E. C.. 1967;The jump of the flea: a study of the energetics and a model of the mechanism. Journal of Experimental Biology 47(1):59–67.Bobbert, M. F.(2001). Dependence of human squat jump performance on the series elastic compliance of the triceps surae: a simulation study. Journal of Experimental Biology, 204(3), 533-542.
Bobbert M. F.. 2001;Dependence of human squat jump performance on the series elastic compliance of the triceps surae: a simulation study. Journal of Experimental Biology 204(3):533–542.Bobbert, M. F., Gerritsen, K. G., Litjens, M. C., & Van Soest, A. J.(1996). Why is countermovement jump height greater than squat jump height? Medicine & Science in Sports and Exercise, 28(11), 1402-1412.
Bobbert M. F., Gerritsen K. G., Litjens M. C., et al, Van Soest A. J.. 1996;Why is countermovement jump height greater than squat jump height? Medicine & Science in Sports and Exercise 28(11):1402–1412. 10.1097/00005768-199611000-00009.Bobbert, M. F., Huijing, P. A., & van Ingen Schenau, G. J.(1986). An estimation of power output and work done by the human triceps surae muscle-tendon complex in jumping. Journal of Biomechanics, 19(11), 899-906.
Bobbert M. F., Huijing P. A., et al, van Ingen Schenau G. J.. 1986;An estimation of power output and work done by the human triceps surae muscle-tendon complex in jumping. Journal of Biomechanics 19(11):899–906. 10.1016/0021-9290(86)90185-5.Bobbert, M. F. & van Ingen Schenau, G. J.(1988). Coordination in vertical jumping. Journal of Biomechanics, 21(3), 249-262.
Bobbert M. F., et al, van Ingen Schenau G. J.. 1988;Coordination in vertical jumping. Journal of Biomechanics 21(3):249–262. 10.1016/0021-9290(88)90175-3.Bohm, H., Cole, G. K., Bruggemann, G. P., & Ruder, H.(2006). Contribution of muscle series elasticity to maximum performance in drop jumping. Journal of Applied Biomechanics, 22(1), 3-13.
Bohm H., Cole G. K., Bruggemann G. P., et al, Ruder H.. 2006;Contribution of muscle series elasticity to maximum performance in drop jumping. Journal of Applied Biomechanics 22(1):3–13.Bosco, C., Tarkka, I., & Komi, P. V.(1982). Effect of elastic energy and myoelectrical potentiation of triceps surae during stretch-shortening cycle exercise. International Journal of Sports Medicine, 3(3), 137-140.
Bosco C., Tarkka I., et al, Komi P. V.. 1982;Effect of elastic energy and myoelectrical potentiation of triceps surae during stretch-shortening cycle exercise. International Journal of Sports Medicine 3(3):137–140. 10.1055/s-2008-1026076.Earp, J. E., Joseph, M., Kraemer, W. J., Newton, R. U., Comstock, B. A., Fragala, M. S., Dunn-Lewis, C., Solomon-Hill, G., Penwell, Z. R., Powell, M. D., Volek, J. S., Denegar, C. R., Hakkinen, K., & Maresh, C. M.(2010). Lower-body muscle structure and its role in jump performance during squat, countermovement, and depth drop jumps. Journal of Strength Conditioning Research, 24(3), 722-729.
Earp J. E., Joseph M., Kraemer W. J., Newton R. U., Comstock B. A., Fragala M. S., Dunn-Lewis C., Solomon-Hill G., Penwell Z. R., Powell M. D., Volek J. S., Denegar C. R., Hakkinen K., et al, Maresh C. M.. 2010;Lower-body muscle structure and its role in jump performance during squat, countermovement, and depth drop jumps. Journal of Strength Conditioning Research 24(3):722–729. 10.1519/jsc.0b013e3181d32c04.Earp, J. E., Kraemer, W. J., Cormie, P., Volek, J. S., Maresh, C. M., Joseph, M., & Newton, R. U.(2011). Influence of muscle-tendon unit structure on rate of force development during the squat, countermovement, and drop jumps. Journal of Strength Conditioning Research, 25(2), 340-347.
Earp J. E., Kraemer W. J., Cormie P., Volek J. S., Maresh C. M., Joseph M., et al, Newton R. U.. 2011;Influence of muscle-tendon unit structure on rate of force development during the squat, countermovement, and drop jumps. Journal of Strength Conditioning Research 25(2):340–347. 10.1519/jsc.0b013e3182052d78.Farris, D. J., Lichtwark, G. A., Brown, N. A., & Cresswell, A. G.(2016). The role of human ankle plantar flexor muscle-tendon interaction and architecture in maximal vertical jumping examined in vivo. Journal of Experimental Biology, 219(4), 528-534.
Farris D. J., Lichtwark G. A., Brown N. A., et al, Cresswell A. G.. 2016;The role of human ankle plantar flexor muscle-tendon interaction and architecture in maximal vertical jumping examined in vivo. Journal of Experimental Biology 219(4):528–534. 10.1242/jeb.126854.Finni, T., Komi, P. V., & Lepola, V.(2000). In vivo human triceps surae and quadriceps femoris muscle function in a squat jump and counter movement jump. European Journal of Applied Physiology, 83(4), 416-426.
Finni T., Komi P. V., et al, Lepola V.. 2000;In vivo human triceps surae and quadriceps femoris muscle function in a squat jump and counter movement jump. European Journal of Applied Physiology 83(4):416–426. 10.1007/s004210000289.Fukashiro, S., Komi, P. V., Jarvinen, M., & Miyashita, M.(1993). Comparison between the directly measured achilles tendon force and the tendon force calculated from the ankle joint moment during vertical jumps. Clinical Biomechanics, 8(1), 25-30.
Fukashiro S., Komi P. V., Jarvinen M., et al, Miyashita M.. 1993;Comparison between the directly measured achilles tendon force and the tendon force calculated from the ankle joint moment during vertical jumps. Clinical Biomechanics 8(1):25–30. 10.1016/s0268-0033(05)80006-3.Gollhofer, A., Strojnik, V., Rapp, W., & Schweizer, L.(1992). Behaviour of triceps surae muscle-tendon complex in different jump conditions. European Journal of Applied Physiology and Occupational Physiology, 64(4), 283-291.
Gollhofer A., Strojnik V., Rapp W., et al, Schweizer L.. 1992;Behaviour of triceps surae muscle-tendon complex in different jump conditions. European Journal of Applied Physiology and Occupational Physiology 64(4):283–291. 10.1007/bf00636213.Hansen, M., Mehler, D., Hessmann, M. H., Blum, J., & Rommens, P. M.(2007). Intramedullary stabilization of extraarticular proximal tibial fractures: a biomechanical comparison of intramedullary and extramedullary implants including a new proximal tibia nail(PTN). Journal of Orthopaedic Trauma, 21(10), 701-709.
Hansen M., Mehler D., Hessmann M. H., Blum J., et al, Rommens P. M.. 2007;Intramedullary stabilization of extraarticular proximal tibial fractures: a biomechanical comparison of intramedullary and extramedullary implants including a new proximal tibia nail(PTN). Journal of Orthopaedic Trauma 21(10):701–709.Hill, A.(1938). The heat of shortening and the dynamic constants of muscle. Proceedings of the Royal Society of London B: Biological Sciences, 126(843), 136-195.
Hill A.. 1938. The heat of shortening and the dynamic constants of muscle. Proceedings of the Royal Society of London B: Biological Sciences 126(843)136–195. 10.1098/rspb.1938.0050.Hodgson, J. A., Finni, T., Lai, A. M., Edgerton, V. R., & Sinha, S.(2006). Influence of structure on the tissue dynamics of the human soleus muscle observed in MRI studies during isometric contractions. Journal of Morphology, 267(5), 584-601.
Hodgson J. A., Finni T., Lai A. M., Edgerton V. R., et al, Sinha S.. 2006;Influence of structure on the tissue dynamics of the human soleus muscle observed in MRI studies during isometric contractions. Journal of Morphology 267(5):584–601. 10.1002/jmor.10421.Hof, A. L., Van Zandwijk, J. P., & Bobbert, M. F.(2002). Mechanics of human triceps surae muscle in walking, running and jumping. Acta Physiologica Scandinavica, 174(1), 17-30.
Hof A. L., Van Zandwijk J. P., et al, Bobbert M. F.. 2002;Mechanics of human triceps surae muscle in walking, running and jumping. Acta Physiologica Scandinavica 174(1):17–30. 10.1046/j.1365-201x.2002.00917.x.Huxley, A. F.(1957). Muscle structure and theories of contraction. Progress in Biophysics and Biophysical Chemistry, 7, 255-318.
Huxley A. F.. 1957;Muscle structure and theories of contraction. Progress in Biophysics and Biophysical Chemistry 7:255–318. 10.1016/s0096-4174(18)30128-8.Huxley, A. F. & Simmons, R. M.(1971). Proposed mechanism of force generation in striated muscle. Nature, 233(5321), 533-538.
Huxley A. F., et al, Simmons R. M.. 1971;Proposed mechanism of force generation in striated muscle. Nature 233(5321):533–538. 10.1038/233533a0.Kiehn, O. & Eken, T.(1997). Prolonged firing in motor units: evidence of plateau potentials in human motoneurons? Journal of Neurophysiology, 78(6), 3061-3068.
Kiehn O., et al, Eken T.. 1997;Prolonged firing in motor units: evidence of plateau potentials in human motoneurons? Journal of Neurophysiology 78(6):3061–3068. 10.1152/jn.1997.78.6.3061.Komi, P. V. & Bosco, C.(1978). Utilization of stored elastic energy in leg extensor muscles by men and women. Medicine & Science in Sports and Exercise, 10(4), 261-265.
Komi P. V., et al, Bosco C.. 1978;Utilization of stored elastic energy in leg extensor muscles by men and women. Medicine & Science in Sports and Exercise 10(4):261–265.Kurokawa, S., Fukunaga, T., & Fukashiro, S.(2001). Behavior of fascicles and tendinous structures of human gastrocnemius during vertical jumping. Journal of Applied Physiology, 90(4), 1349-1358.
Kurokawa S., Fukunaga T., et al, Fukashiro S.. 2001;Behavior of fascicles and tendinous structures of human gastrocnemius during vertical jumping. Journal of Applied Physiology 90(4):1349–1358. 10.1152/jappl.2001.90.4.1349.Lee, H. D., Finni, T., Hodgson, J. A., Lai, A. M., Edgerton, V. R., & Sinha, S.(2006). Soleus aponeurosis strain distribution following chronic unloading in humans: an in vivo MR phase-contrast study. Journal of Applied Physiology, 100(6), 2004-2011.
Lee H. D., Finni T., Hodgson J. A., Lai A. M., Edgerton V. R., et al, Sinha S.. 2006;Soleus aponeurosis strain distribution following chronic unloading in humans: an in vivo MR phase-contrast study. Journal of Applied Physiology 100(6):2004–2011. 10.1152/japplphysiol.01085.2005.Linke, W. A.(2000). Titin elasticity in the context of the sarcomere: force and extensibility measurements on single myofibrils. Advances in Experimental Medicine and Biology, 481, 179-202.
Linke W. A.. 2000;Titin elasticity in the context of the sarcomere: force and extensibility measurements on single myofibrils. Advances in Experimental Medicine and Biology 481:179–202.Maganaris, C. N. & Paul, J. P.(2002). Tensile properties of the in vivo human gastrocnemius tendon. Journal of Biomechanics, 35(12), 1639-1646.
Maganaris C. N., et al, Paul J. P.. 2002;Tensile properties of the in vivo human gastrocnemius tendon. Journal of Biomechanics 35(12):1639–1646. 10.1016/s0021-9290(02)00240-3.Magnusson, S. P., Aagaard, P., Dyhre-Poulsen, P., & Kjaer, M.(2001). Load-displacement properties of the human triceps surae aponeurosis in vivo. Journal of Physiology, 531(1), 277-288.
Magnusson S. P., Aagaard P., Dyhre-Poulsen P., et al, Kjaer M.. 2001;Load-displacement properties of the human triceps surae aponeurosis in vivo. Journal of Physiology 531(1):277–288. 10.1111/j.1469-7793.2001.0277j.x.Marsh, R. L.(1994). Jumping ability of anuran amphibians. Advances in Veterinary Science and Comparative Medicine, 38B, 51-111.
Marsh R. L.. 1994;Jumping ability of anuran amphibians. Advances in Veterinary Science and Comparative Medicine 38B:51–111.Marsh, R. L.(1999). How muscles deal with real-world loads: the influence of length trajectory on muscle performance. Journal of Experimental Biology, 202(23), 3377-3385.
Marsh R. L.. 1999;How muscles deal with real-world loads: the influence of length trajectory on muscle performance. Journal of Experimental Biology 202(23):3377–3385.Peplowski, M. M. & Marsh, R. L.(1997). Work and power output in the hindlimb muscles of Cuban tree frogs Osteopilus septentrionalis during jumping. Journal of Experimental Biology, 200(22), 2861-2870.
Peplowski M. M., et al, Marsh R. L.. 1997;Work and power output in the hindlimb muscles of Cuban tree frogs Osteopilus septentrionalis during jumping. Journal of Experimental Biology 200(22):2861–2870.Roberts, T. J.(2016). Contribution of elastic tissues to the mechanics and energetics of muscle function during movement. Journal of Experimental Biology, 219(2), 266-275.
Roberts T. J.. 2016;Contribution of elastic tissues to the mechanics and energetics of muscle function during movement. Journal of Experimental Biology 219(2):266–275. 10.1242/jeb.124446.Roberts, T. J. & Azizi, E.(2011). Flexible mechanisms: the diverse roles of biological springs in vertebrate movement. Journal of Experimental Biology, 214(3), 353-361.
Roberts T. J., et al, Azizi E.. 2011;Flexible mechanisms: the diverse roles of biological springs in vertebrate movement. Journal of Experimental Biology 214(3):353–361. 10.1242/jeb.038588.Roberts, T. J. & Marsh, R. L.(2003). Probing the limits to muscle-powered accelerations: lessons from jumping bullfrogs. Journal of Experimental Biology, 206(15), 2567-2580.
Roberts T. J., et al, Marsh R. L.. 2003;Probing the limits to muscle-powered accelerations: lessons from jumping bullfrogs. Journal of Experimental Biology 206(15):2567–2580. 10.1242/jeb.00452.Spagele, T., Kistner, A., & Gollhofer, A.(1999). Modelling, simulation and optimisation of a human vertical jump. Journal of Biomechanics, 32(5), 521-530.
Spagele T., Kistner A., et al, Gollhofer A.. 1999;Modelling, simulation and optimisation of a human vertical jump. Journal of Biomechanics 32(5):521–530.Svantesson, U., Grimby, G., & Thomee, R.(1994). Potentiation of concentric plantar flexion torque following eccentric and isometric muscle actions. Acta Physiologica Scandinavica, 152(3), 287-293.
Svantesson U., Grimby G., et al, Thomee R.. 1994;Potentiation of concentric plantar flexion torque following eccentric and isometric muscle actions. Acta Physiologica Scandinavica 152(3):287–293. 10.1111/j.1748-1716.1994.tb09808.x.Wakabayashi, K., Sugimoto, Y., Tanaka, H., Ueno, Y., Takezawa, Y., & Amemiya, Y.(1994). X-ray diffraction evidence for the extensibility of actin and myosin filaments during muscle contraction. Biophysical Journal, 67(6), 2422.
Wakabayashi K., Sugimoto Y., Tanaka H., Ueno Y., Takezawa Y., et al, Amemiya Y.. 1994;X-ray diffraction evidence for the extensibility of actin and myosin filaments during muscle contraction. Biophysical Journal 67(6):2422. 10.1016/s0006-3495(94)80729-5.Wang, J. H.(2006). Mechanobiology of tendon. Journal of Biomechanics, 39(9), 1563-1582.
Wang J. H.. 2006;Mechanobiology of tendon. Journal of Biomechanics 39(9):1563–1582. 10.1016/j.jbiomech.2005.05.011.Zajac, F. E.(1993). Muscle coordination of movement: a perspective. Journal of Biomechanics, 26(1), 109-124.
Zajac F. E.. 1993;Muscle coordination of movement: a perspective. Journal of Biomechanics 26(1):109–124. 10.1016/0021-9290(93)90083-q.